המקומות הטובים
יוזמה אומנותית חדשה מציגה את מרחב הטכניון באמצעות חוויותיהם האישיות של 16 סטודנטיות וסטודנטים מהעולם
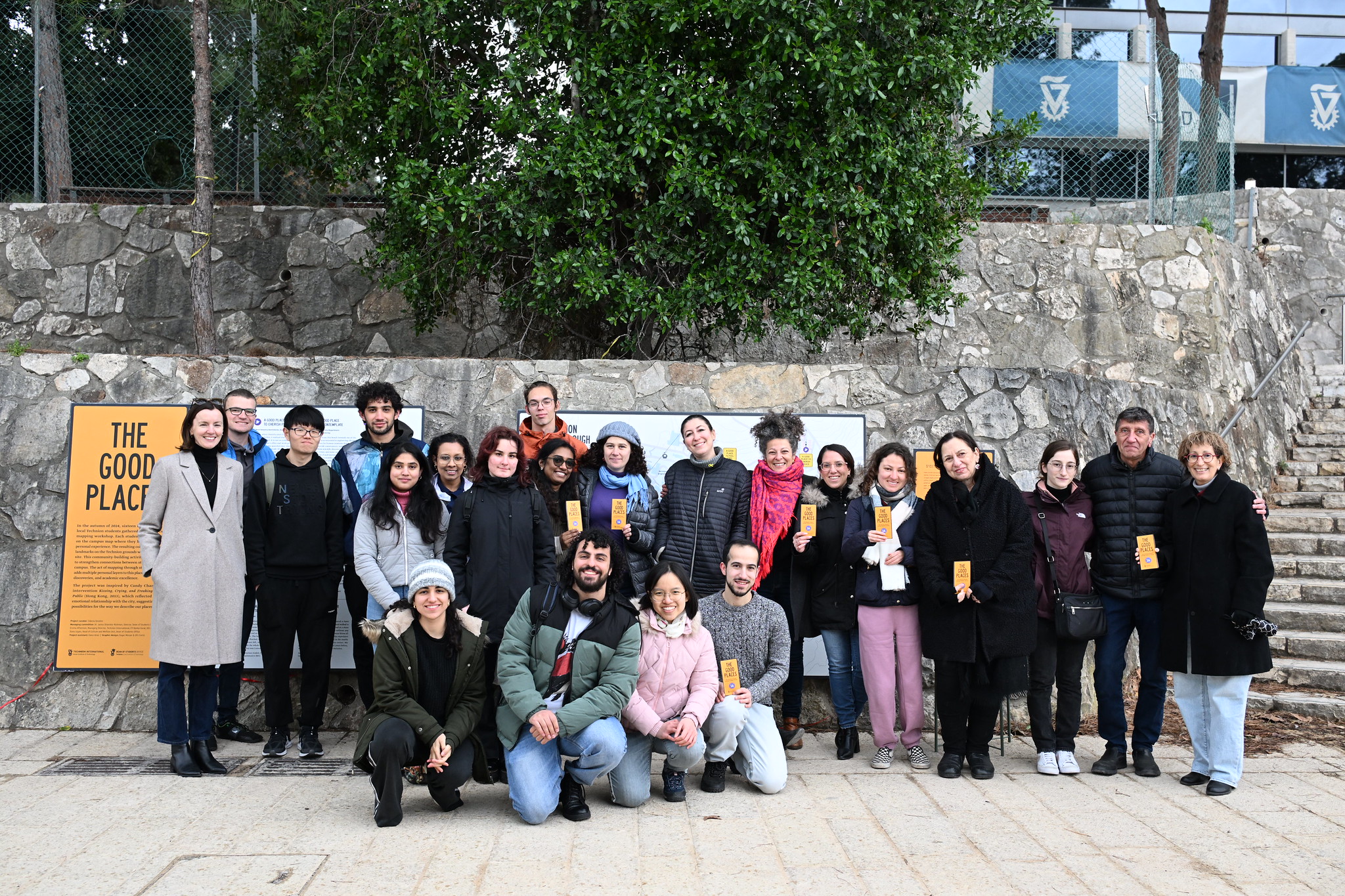
יוזמה אומנותית חדשה מציגה את מרחב הטכניון באמצעות חוויותיהם האישיות של 16 סטודנטיות וסטודנטים מהעולם
Technion VC Club הוא מועדון יזמות והון-סיכון המחבר בין מצוינות אקדמית לעולם היזמות וההון סיכון
גישה חדשנית המדמה מערכת הראייה של חרקים שפותחה בטכניון צפויה להשפיע על ניטור תהליכים מולקולריים של סרטן ומחלות אחרות
חברת הכנסת קטי שטרית, החברה בוועדת החינוך, התרבות והספורט, ביקרה אתמול לראשונה בטכניון ונפגשה עם נשיא הטכניון פרופ' אורי סיון והמשנה הבכיר לנשיא פרופ' עודד רבינוביץ'.
100000
בוגרים
18
פקולטות
15000
סטודנטים
60
מרכזי מחקר
ברחבי הקמפוס